In the midst of a pandemic that has brought so much worry and loss, it’s natural to want to help — to do some small part to solve a problem, to counter pain, or to, importantly, remind others that there is beauty and wonder in the world. Scientists have long been doing just that. Many are chasing answers to the myriad challenges that people face every day, and revealing the rewards in the pursuit of knowledge itself. It’s in that spirit that we present this year’s SN 10: Scientists to Watch.
For the sixth consecutive year, Science News is featuring 10 early- and mid-career scientists who are pushing the boundaries of scientific inquiry. Some of the researchers are asking questions with huge societal importance: How do we prevent teen suicide? What are the ingredients in wildfire smoke that are damaging to health? Is there a better way to monitor earthquakes to save lives? What about finding new ways to diagnose and treat diseases?
Others are trying to grasp how weird and wonderful the natural world is — from exploring how many supermassive black holes are out there in space to understanding the minuscule genetic details that drive evolution. For instance, SaraH Zanders, one of this year’s SN 10, is unveiling the drama that unfolds when life divvies up its genetic material.
A couple of the scientists on this year’s list have also taken steps to support people from groups that are underrepresented in the sciences. These researchers see how science benefits when people from diverse backgrounds contribute to the pursuit of answers.
All of this year’s honorees are age 40 and under, and all were nominated by Nobel laureates, recently elected members of the U.S. National Academy of Sciences or previous SN 10 scientists. The world feels very different than it did at the start of 2020, when we first put out our call for SN 10 nominations, but the passion these scientists have for their work endures. The curiosity, creativity and drive of this crew offers hope that we can overcome some of our biggest challenges.
Though it often takes time, out of crisis comes action. Also out of crisis comes a renewed appreciation for small pleasures that give life meaning. These researchers find joy in the search for scientific answers. Here’s how Zanders describes what motivates her work: “It’s just I like to solve puzzles.” — Elizabeth Quill
The 2020 SN 10: Scientists to Watch
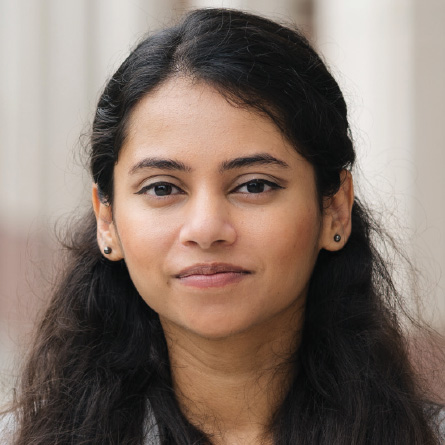
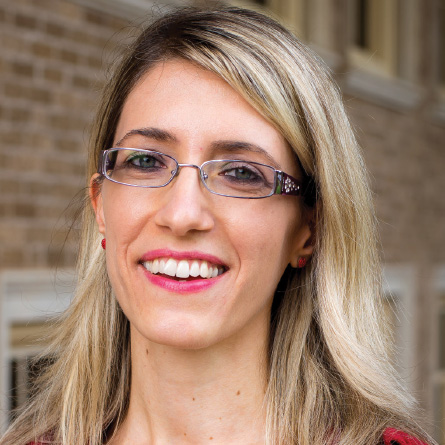
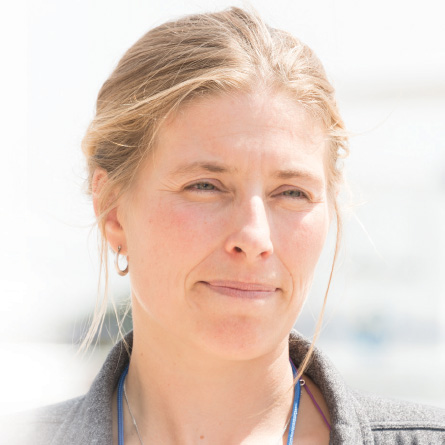
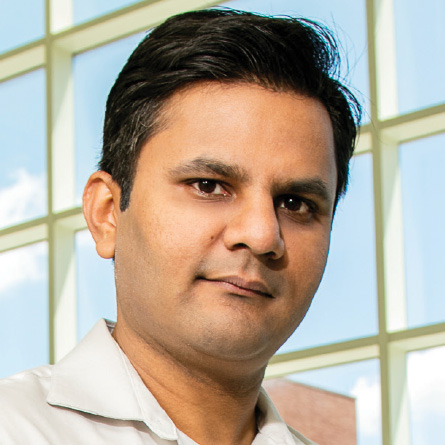
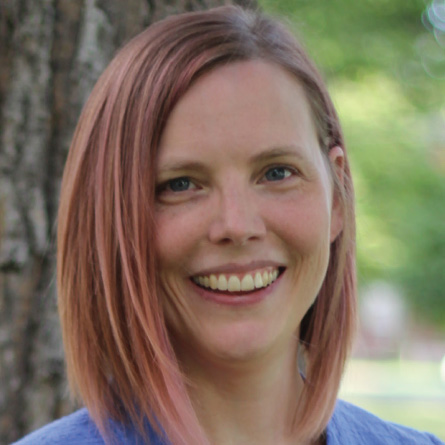
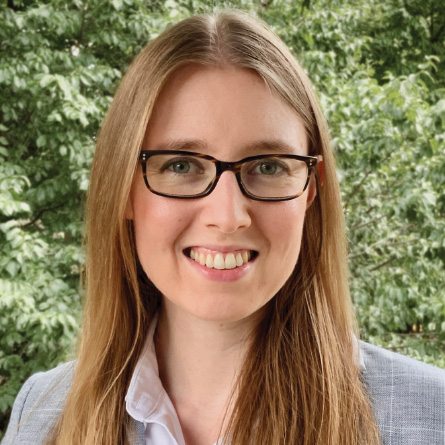
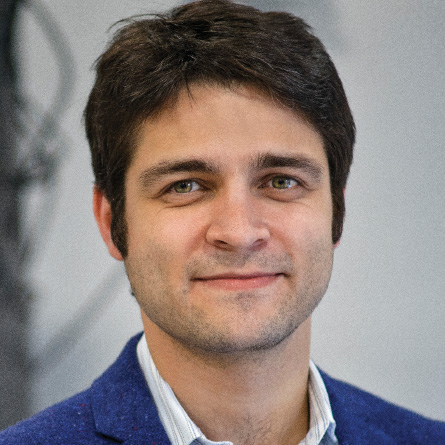
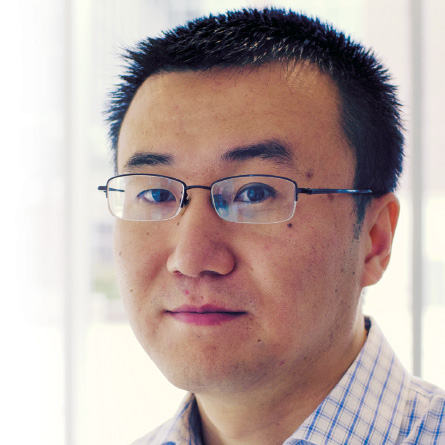
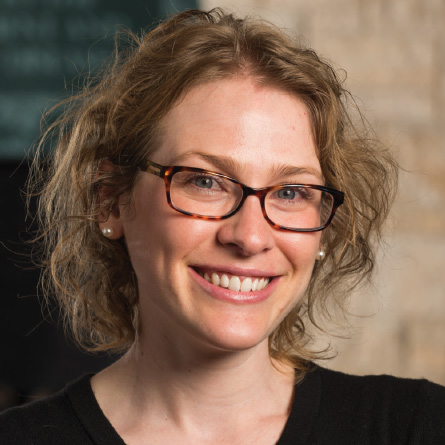
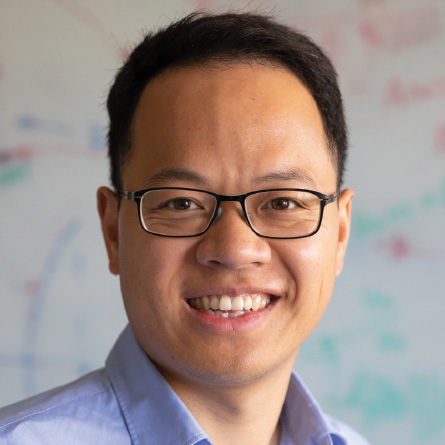
Black hole hunter seeks a cosmic census
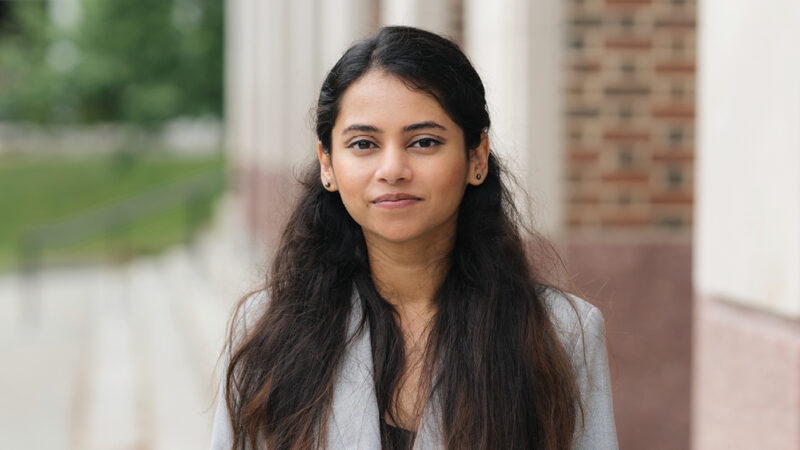
Tonima Tasnim Ananna, 29Astrophysicist
Affiliation: Dartmouth CollegeHometown: Dhaka, BangladeshFavorite black hole: Cygnus X-1
Standout research
Tonima Tasnim Ananna is bringing the heaviest black holes out of hiding. She has drawn the most complete picture yet of black holes across the universe — where they are, how they grow and how they affect their environments. And she did it with the help of artificial intelligence.
As far as astronomers can tell, nearly every galaxy stows a black hole at its center, weighing millions or billions of times the mass of the sun. Though these supermassive black holes can heat surrounding material until it glows brighter than all the galaxy’s stars combined, the light can be concealed by gas and dust also drawn in by the black hole’s pull. High-energy X-rays cut through that dusty veil. So for her Ph.D., completed in 2019, Ananna gathered surveys from four X-ray telescopes, more datasets than any previous study had used. Her goal was to create a model of how black holes grow and change across cosmic history. “It was supposed to be a short paper,” Ananna says. But models that explained one or a few of the datasets didn’t work for the full sample. “It stumped us for some time.”
To break the gridlock, she developed a neural network, a type of artificial intelligence, to find a description of the black hole population that explained what all the observatories saw. “She just went off and taught herself machine learning,” says astrophysicist Meg Urry of Yale University, Ananna’s Ph.D. adviser. “She doesn’t say, ‘Oh, I can’t do this.’ She just figures out a way to learn it and do it.” One early result of the model suggests that there are many more active black holes out there than previously realized.
Big goal
Black holes could be gobbling down gas as fast as theoretically possible.
Galaxies live and die by their black holes. “When a black hole puts out energy into the galaxy, it can cause stars to form,” Ananna says. “Or it could blow gas away,” shutting down star formation and stunting the galaxy’s growth (SN: 3/31/20). So understanding black holes is key to understanding how cosmic structures — everything from galaxy clusters down to planets and perhaps even life — came to be. Ananna’s model is built on data describing black holes at different cosmic distances. Because looking far in space is like looking back in time, the model shows how black holes grow and change over time. It could also help figure out how efficiently black holes eat. Early hints suggest black holes could be gobbling down gas as fast as theoretically possible, which may help explain how some got so big so fast (SN: 3/16/18).
Inspiration
When Ananna was a 5-year-old in Dhaka, Bangladesh, her mother told her about the Pathfinder spacecraft landing on Mars. Her mother was a homemaker, she says, but was curious about science and encouraged Ananna’s curiosity, too. “That’s when I realized there were other worlds,” she says. “That’s when I wanted to study astronomy.” There were not a lot of opportunities to study space in Bangladesh, so she came to the United States for undergrad, attending Bryn Mawr College in Pennsylvania. She chose an all-women’s school not known for a lot of drinking to reassure her parents that she was not “going abroad to party.” Although Ananna intended to keep her head down and study, she was surprised by the social opportunities she found. “The women at Bryn Mawr were fiercely feminist, articulate, opinionated and independent,” she says. “It really helped me grow a lot.” Traveling for internships at NASA and CERN, the European particle physics laboratory near Geneva, and a year at the University of Cambridge, boosted her confidence. (She did end up going to some parties — “no alcohol for me, though.”)
Now, Ananna is giving back. She cofounded Wi-STEM (pronounced “wisdom”), a mentorship network for girls and young women who are interested in science. She and four other Bangladeshi scientists who studied in the United States mentor a group of 20 female high school and college students in Bangladesh, helping them find paths to pursue science. — Lisa Grossman
Pioneer pairs light with gravity waves
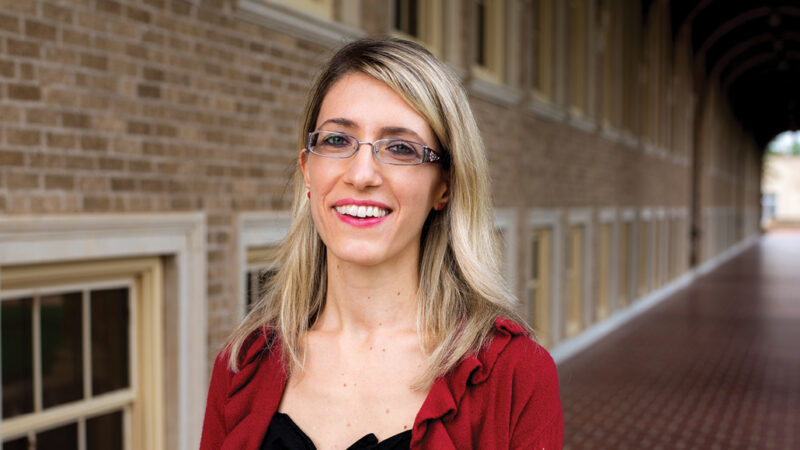
Alessandra Corsi, 40Astrophysicist
Affiliation: Texas Tech UniversityHometown: Rome, ItalyFavorite telescope: Very Large Array, New Mexico
Standout research
On September 3, 2017, Alessandra Corsi finally saw what she had been waiting for since mid-August: a small dot in her telescope images that was the radio afterglow of a neutron star collision. That stellar clash, discovered by the Advanced Laser Interferometer Gravitational-Wave Observatory team, or LIGO, which included Corsi, was the first direct sighting of a neutron star collision (SN: 10/16/17). The event, dubbed GW170817, was also the first of any kind seen in both gravitational waves and light waves.
Telescopes around the world spotted all kinds of light from the crash site, but one particular kind, the radio waves, took their sweet time showing up. Corsi had been waiting since August 17, when the gravitational waves were spotted. “Longest two weeks of my life,” Corsi says. The radio waves were key to understanding a superfast particle jet launched by the colliding stars.
Early on, the jet appeared to have been smothered by a plume of debris from the collision (SN: 12/20/17). But follow-up radio observations made by Corsi’s team and others confirmed that the jet had punched through the wreckage (SN: 2/22/19). This jet was the first of its kind to be seen from the side, allowing Corsi and colleagues to probe its structure. The jet almost certainly would have gone unnoticed if the gravitational waves hadn’t clued astronomers in.
Big goal
Corsi is a pioneer in the new field of multimessenger astronomy, which pairs observations of light waves with spacetime ripples, or gravitational waves. The pairing is like having eyes and ears on the cosmos, Corsi says. “You cannot learn all that you could with only one of the two.” In the case of GW170817, gravitational waves revealed how the neutron stars danced around each other as they spiraled toward collision, and light waves unveiled the type of material left in the aftermath (SN: 10/23/19). Using this multimessenger approach could also give astronomers a more complete picture of other cataclysms, such as smashups between neutron stars and black holes, and the explosive deaths of massive stars. Such spectacular events “reveal some of the most fundamental physics in our universe,” Corsi says.
If gravitational wave signals were converted into sound, they would create their own kind of music.
Most researchers specialize in either gravitational waves or light, but Corsi “is very well-versed in both messengers,” says Wen-fai Fong, an astrophysicist at Northwestern University in Evanston, Ill. “That makes her extremely versatile in terms of the types of multimessenger science she can study.”
What’s next
Corsi has now built a computational tool to scan LIGO data for gravitational waves stirred up by whatever is left behind in a neutron star merger. The tool is based on a paper she published in 2009 — years before LIGO scored its first gravitational wave detection (SN: 2/11/16). The paper describes the gravitational wave pattern that would signal the presence of one possible remnant: a rapidly spinning, elongated neutron star. Alternatively, a neutron star smashup could leave behind a black hole. Knowing which “tells us a lot about how matter behaves at densities way higher than we could ever explore in a lab,” Corsi says.
Inspiration
Corsi taught herself to play the piano in high school, and now enjoys playing both classical music and tunes from favorite childhood movies, like Beauty and the Beast. The audio frequencies of piano notes are similar to the frequencies of spacetime tremors picked up by LIGO. If gravitational wave signals were converted into sound, they would create their own kind of music. “That’s the thing I like to think of when I’m playing,” she says. — Maria Temming
What’s in smoky air?
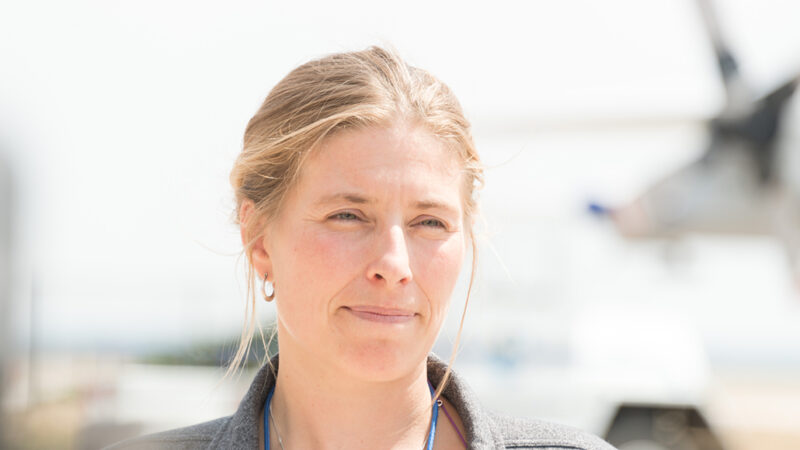
Emily Fischer, 39Atmospheric chemist
Affiliation: Colorado State UniversityHometown: Richmond, R.I.Favorite outdoor activities: Cross-country skiing and gardening
Motivation
Emily Fischer has always cared about air pollution. “It’s innate.… It’s a calling,” she says. Exposure to air pollution raises your risk for many common ailments, such as cardiovascular disease, asthma, diabetes and obesity. But unlike some other risk factors for these diseases, “you can’t choose not to breathe, right? You have to have clean air for everyone.” In her youth, she organized rallies to clean up the cigarette smoke–filled air of her Rhode Island high school. That interest led Fischer to study atmospheric chemistry and motivates her current work as a self-described air pollution detective. Air pollution may conjure images of thick black plumes billowing from smokestacks, but Fischer says most air pollution is invisible and poorly understood. She combines analytical chemistry with high-flying techniques to understand where air pollution comes from and how it changes as it moves through the air.
Bold idea
Wildfire smoke like that filling the skies in the American West this season is a major, but still mysterious, source of air pollution. Thousands of different solids, liquids and gases swirl together to form wildfire smoke, and its chemical composition changes as it blows through the atmosphere. This dynamic mixture, which is also affected by what’s burning on the ground, is tricky to measure, since each of its many components requires highly specialized equipment and expertise to assess. The equipment also has to be airborne, typically lofted into the air via planes or balloons. “There has been beautiful work on wildfire smoke,” Fischer says, “but in most studies, we just have not had all the measurements needed to really interpret things.”
“You can’t choose not to breathe, right? You have to have clean air for everyone.”
Emily Fischer
To get a fuller view, she dreamed big: “Why not try to measure everything, and measure it systematically?” She pulled together a diverse team of 10 lead researchers, and scores more graduate students and postdocs, to pull off the most comprehensive analysis of wildfire smoke ever attempted, a project dubbed WE-CAN. During the summer of 2018, Fischer led over a dozen six-hour flights over the West, chasing wildfire smoke plumes and systematically measuring the air in and around smoke plumes with nearly 30 different instruments crammed into the cargo hold of a C-130 plane.
“[WE-CAN] is a big collaboration,” says Ronald Cohen, an atmospheric chemist at the University of California, Berkeley. He says success stemmed in large part from the team that came together.
“Making an environment for successful collaboration is really satisfying to me,” Fischer says.
While team members are still analyzing the data, the project is already revealing some of the smoke’s secrets. For example, formaldehyde and hydrogen cyanide — two chemicals linked to cancer and other health problems — are abundant in wildfire smoke. Recent wildfires show how important it is to understand the role of climate change in fires, Fischer says, and “who is most vulnerable in our society, and how we can best prepare and protect those communities.”
Fisher is also planning to adapt some of what she’s learned from WE-CAN to track ammonia emissions from farms and feed lots, which are another major source of air pollution.
Big goal
Fischer is deeply committed to bringing more undergraduate women, especially women of color, into the geosciences. And she’s using science to figure out how. She brought a team of social scientists and geoscientists together to study how different interventions can help. She and colleagues found that for every female role model a student has, her probability of continuing on in her geosciences major roughly doubles. Having someone to look up to who looks like them is key to building a sense of belonging and identity as a scientist, Fischer says. To help build that network, Fischer started PROGRESS, a workshop and mentorship program that aims to support undergraduate women in the geosciences. Started at Colorado State University in 2014, the program has since expanded, reaching over 300 women at institutions across the United States.
For her own mentees, Fischer tries to instill a willingness to take risks and go after big, bold questions. “The easy things are done,” she says. Pushing forward our understanding of pressing questions means chasing research projects that might lead nowhere, she says, or might crack open a new field of research. “It’s OK to be wrong, and it’s OK to take risks. That’s what science needs right now.” — Jonathan Lambert
Taking chemistry lessons from nature
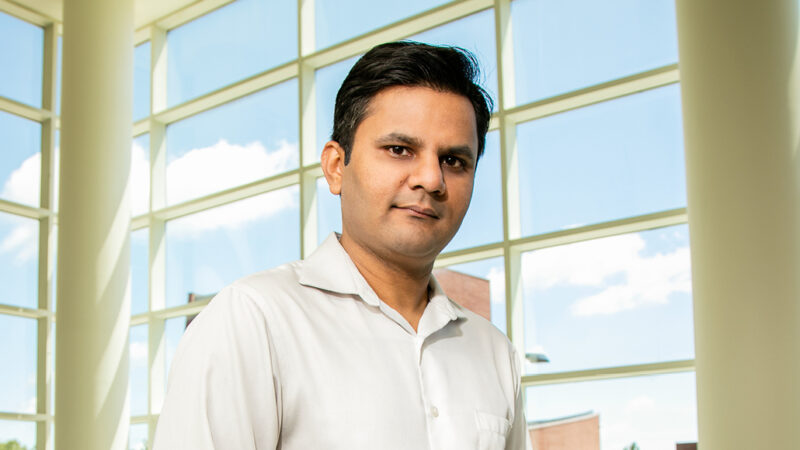
Prashant Jain, 38Physical chemist
Affiliation: University of Illinois at Urbana-ChampaignHometown: Mumbai, IndiaFavorite element: Gold
Big goal
Prashant Jain explores how light interacts with matter — such as how plants use sunlight to photosynthesize — and applies that knowledge to new problems. He recently took lessons from nature to convert carbon dioxide into other useful molecules. In a paper last year in Nature Communications, Jain and Sungju Yu, also at Illinois at the time, reported using gold nanoparticles as a catalyst to drive chemical reactions between carbon dioxide and water.
When light hit the nanoparticles, it set off a series of reactions that converted carbon dioxide into hydrocarbon fuels such as methane and propane. In essence, the process not only sucked carbon dioxide — a greenhouse gas — out of the air, but it also made that carbon into fuel. No wonder the oil giant Shell is funding Jain’s work. The whole process isn’t very efficient, so Jain is working to improve how much carbon dioxide gets used and how much fuel gets produced. But along the way he hopes to learn more about how nature uses energy to make matter — and to inspire his lab to create more sustainable and renewable energy technologies.
“I am myself still a student.”
Prashant Jain
In another example of using chemistry to push toward future technologies, Jain and colleagues shined light on gold and platinum nanoparticles and triggered reactions that liberated hydrogen from ammonia molecules. Hydrogen is important in many industries — fuel cells for zero-carbon vehicles use it, for example — but it can be dangerous to transport because it’s flammable. Jain’s discovery could allow workers to transport ammonia instead, which is safer, and then free the hydrogen from the ammonia once it has arrived where’s it needed. The work was reported online in July in Angewandte Chemie.
Superpower
Jain has a remarkable ability and optimism to see unsuccessful laboratory experiments as successful steps toward understanding the natural world, says Karthish Manthiram, a chemical engineer at MIT. As a first-year graduate student at the University of California, Berkeley, Manthiram remembers being frustrated that his experiments weren’t turning out as expected. But Jain, a postdoctoral fellow in the same lab, stepped in to help and recast the problematic results. “He’s always viewed what others see as failure as moments of clarity that build up to moments when things make more sense,” Manthiram says. “For me that was an important lesson in how to be a scientist.”
Inspiration
Growing up in a family that worked mostly in business and finance, Jain fell in love with science as a preteen — inspired in part by watching the movie Jurassic Park and its fictional depiction of what might be possible through understanding the molecular world. Soon he spotted a physics textbook for sale from a street vendor and bought it. “I tried to read the book, nothing much made sense,” he says. “I wanted to be the one to figure out all these mysteries of nature.” He chose to major in chemical engineering in college (inspired in part by a magazine published by the chemical company DuPont), and then switched to physical chemistry when he moved to the United States to get a Ph.D.
Promoted this year to full professor, Jain has never stopped pushing to acquire new knowledge; when he finished teaching this last spring semester, he enrolled in an online MIT course on quantum information science. “I am myself still a student,” he says. — Alexandra Witze
Challenging ideas about youth suicide
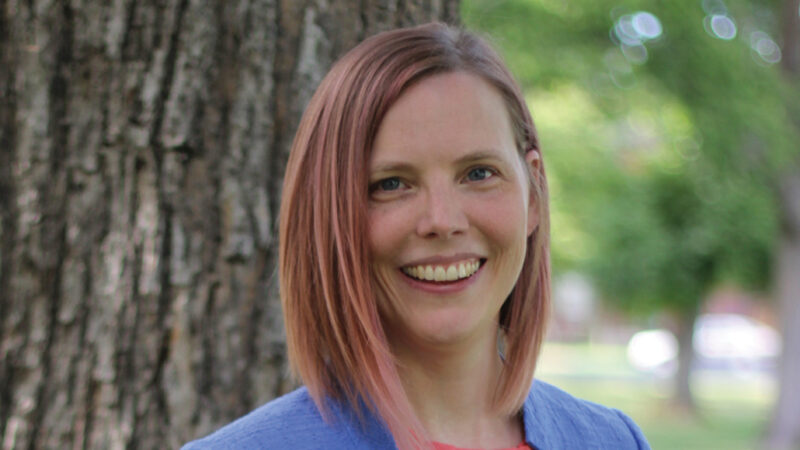
Anna Mueller, 40Sociologist
Affiliation: Indiana UniversityHometown: Houston, TexasFavorite fieldwork: Observing rituals
Standout research
Between 2000 and 2015, at a high school of about 2,000 students in the town of Poplar Grove (a pseudonym), 16 former and current students died by suicide; three other similar-aged individuals in the community, mostly at private schools, also took their own lives. A clinician who had grown up in the town reached out to Anna Mueller for help breaking the cruel cycle. Before that e-mail in fall 2013, Mueller was using big data to understand why teen and young adult suicide rates in the United States were spiking. The U.S. Centers for Disease Control and Prevention estimates that suicides among 10- to 24-year-olds jumped 56 percent between 2007 and 2017.
Scholars theorized that suicidal people attracted other suicidal people. But Mueller’s work undercut that idea. In 2015 in the Journal of Health and Social Behavior, for instance, she reported that merely having a suicidal friend did not increase a teen’s suicide risk. A teen’s risk only went up with awareness that a teenage friend had made a suicide attempt. “Knowledge of the attempt matters to transforming … risk,” Mueller says. She carried an understanding of that contagion effect to Poplar Grove, where she worked with sociologist Seth Abrutyn of the University of British Columbia in Vancouver, the half of the duo who is more focused on the theoretical.
Anna Mueller’s long-term goal is to create a sort of litmus test that identifies schools that could be at risk of a suicide cluster.
The team conducted 110 interviews and focus group meetings, lasting from 45 minutes to four hours, with Poplar Grove residents, plus some individuals outside the community for comparison. The team’s research revealed that teens felt an intense pressure to achieve in their affluent, mostly white town, where everybody seemed to know everyone else. While teens and young adults in a first wave of suicides might have had mental health problems, peers and community members often attributed those deaths to the town’s pressure cooker environment. That narrative, however incomplete, was especially strong when the youth who killed themselves were classic overachievers. Tragically, over time, that script became embedded in the local culture, making even youth who weren’t previously suicidal see suicide as a viable option (SN: 4/3/19), Mueller says.
Mueller and Abrutyn were among the first researchers to start chipping away at the underlying reasons for why suicide rates have been rising in high schoolers, particularly overachieving girls without obvious underlying mental health problems, says Bernice Pescosolido, a sociologist at Indiana University in Bloomington who helped bring Mueller into the school’s sociology department. “What Anna and Seth have really been able to show is how imitation works and what the contagion effect looks like on the ground.”
Big goal
Mueller’s long-term goal is to create a sort of litmus test that identifies schools that could be at risk of a suicide cluster. That way, school and community leaders can intervene before the first suicide and its resulting firestorm. Since fall 2018, she has been researching suicide trends in school districts in Colorado that are more diverse than Poplar Grove. When it comes to school culture, her early work shows, there’s often a trade-off between academic or athletic excellence and a supportive environment.
Top tool
In anticipation of her work in Poplar Grove, Mueller knew she needed a more boots-on-the-ground approach than her big data training allowed. So she trained in qualitative methods, including how to design a study; interview techniques, such as how to write questions to elicit desired conversations; and the detailed data analysis required for this research tactic.
Mueller also sees the value in observing interactions, a common sociological approach. This spring, with the pandemic in full swing, she spent a lot of time on her home computer watching socially distant graduation ceremonies in her Colorado schools. She found that a school’s culture showed in the details, such as whether valedictorians addressed hot-button issues, such as the Black Lives Matter movement, in their speeches. “Of all of my moments in the field, rituals are the ones that tug at my own heartstrings because I’m watching kids graduate and that’s just inherently beautiful, but it also is a very powerful data moment,” she says. — Sujata Gupta
The National Suicide Prevention Lifeline can be reached at 1-800-273-TALK (8255).
The inner lives of protons and neutrons
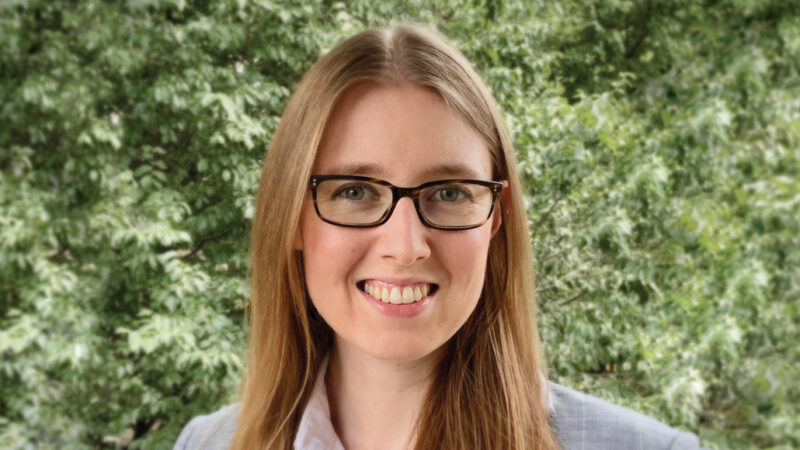
Phiala Shanahan, 29Theoretical physicist
Affiliation: MITHometown: Adelaide, AustraliaFavorite subatomic particle: The gluon
Big goal
When Phiala Shanahan was a graduate student, she was shocked to learn that experiments disagreed on the size of the proton (SN: 9/10/19). “Protons and neutrons are the key building blocks of 99 percent of the visible matter in the universe,” she says. “And we know, in some sense, surprisingly little about their internal structure.”
“If there’s something I don’t understand, I’m extremely stubborn when it comes to figuring out the answer.”
Phiala Shanahan
That ignorance inspires her studies. She aims to calculate the characteristics of protons and neutrons based on fundamental physics. That includes not just their size, but also their mass and the nature of their components — how, for example, the quarks and gluons that make them up are sprinkled around inside. Such calculations can help scientists put the standard model, the theory that governs elementary particles and their interactions, to the test.
Standout research
Shanahan is known for her prowess calculating the influence of gluons, particles that carry the strong force, which binds the proton together. For example, when gluons’ contributions are included, the proton is squeezed to a pressure greater than estimated to exist within incredibly dense neutron stars, she and a coauthor reported in Physical Review Letters in 2019. “It’s a very remarkable calculation,” says physicist Volker Burkert of the Thomas Jefferson National Accelerator Facility in Newport News, Va. “That’s very fundamental, and it’s the first time it has been done.” Because they have no electric charge, gluons tend to elude experimental measurements, and that has left the particles neglected in theoretical calculations as well. Shanahan’s gluon results should be testable at a new particle collider, the Electron-Ion Collider, planned to be built at Brookhaven National Lab in Upton, N.Y. (SN: 4/18/17).
Superpower
Persistence. “I hate not knowing something,” she says. “So if there’s something I don’t understand, I’m extremely stubborn when it comes to figuring out the answer.”
Top tool
A technique called lattice QCD is the foundation for Shanahan’s work. It’s named for quantum chromodynamics, the piece of the standard model that describes the behavior of quarks and gluons. QCD should allow scientists to predict the properties of protons and neutrons from the bottom up, but the theory is incredibly complex, making full calculations impossible to perform even on the best available supercomputers. Lattice QCD is a shortcut. It breaks up space and time into a grid on which particles reside, simplifying calculations. Shanahan is leading efforts to use machine learning to rev up lattice QCD calculations — putting her persistence to good use. “We don’t have to rely on computers getting better. We can have smarter algorithms for exploiting those computers,” she says. She hopes to speed up calculations enough that she can go beyond protons and neutrons, working her way up to the properties of atomic nuclei. — Emily Conover
How to engineer cellular helpers
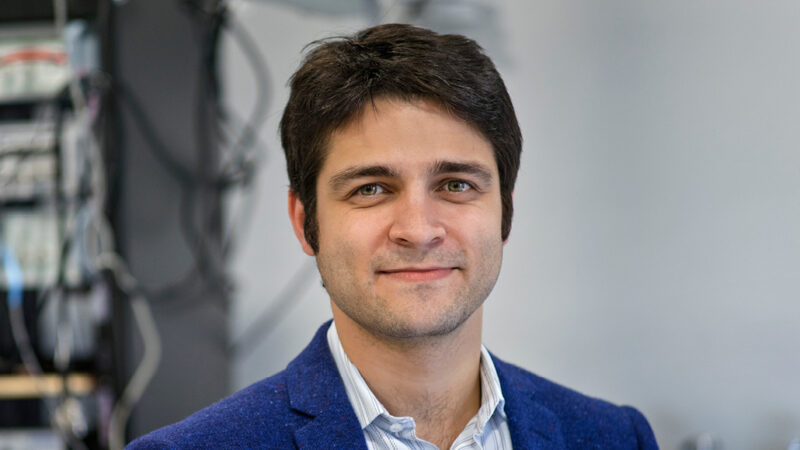
Mikhail Shapiro, 39Biochemical engineer
Affiliation: CaltechHometown: Kolomna, RussiaFavorite protein: He can’t pick just one
Bold idea
Mikhail Shapiro believes that in the future, “we’re going to have smart biological devices that are roaming our bodies, diagnosing and treating disease” — something akin to the submarine in the 1966 classic sci-fi film Fantastic Voyage. As the shrunken sub entered and repaired the body of a sick scientist, commanders on the outside helped control it. “Similarly, we’re going to want to talk to the cells that we are going to send into the body to treat cancer, or inflammation, or neurological diseases,” Shapiro says.
Shapiro and his colleagues are working on building, watching and controlling such cellular submarines in the real world. Such a deep view inside the body might offer clues to basic science questions, such as how communities of gut bacteria grow, how immune cells migrate through the body or how brains are built cell by cell.
Despite his futuristic visions, Shapiro is often drawn to the past. “I like science history a lot,” he says. Right now, he’s in the middle of rereading the Pulitzer Prize–winning The Making of the Atomic Bomb. Just before that, he read a biography of Marie Curie.
Standout research
“There is not a protein that I learn about that I don’t think about ways to misuse it,” Shapiro says. But he’s especially fond of the proteins that build the outer shell of gas vesicles in certain kinds of bacteria. These microscopic air bags “have so many uses that were totally unanticipated,” Shapiro says.
In addition to letting bacteria sink or float, these bubbles provide a communication system, Shapiro and colleagues have found. Over the last several years, they have coaxed both bacterial cells and human cells to make gas vesicles and have placed such cells within mice. Because the air-filled pockets reflect sound, the engineered cells can be tracked from outside a mouse’s body. Using patterns of sound waves, the researchers can also drive bacterial cells around in lab dishes.
“There is not a protein that I learn about that I don’t think about ways to misuse it.”
Mikhail Shapiro
In another nod to Fantastic Voyage, scientists can weaponize these cellular submarines. “We’ve essentially turned cells into suicide agents triggered by ultrasound,” Shapiro says. This explosion could release chemicals into the surroundings and destroy nearby cells. This sort of targeted detonation could be damaging to tumors, for instance. “Complete warfare is possible,” he says.
By seeing the potential in these esoteric gas vesicles, Shapiro was “ahead of his time and hugely innovative,” says Jason Lewis, a molecular imaging scientist at Memorial Sloan Kettering Cancer Center in New York City. “I think we’ve only scratched the surface of what his work will do in terms of a greater impact.”
Motivation
“Frustration,” Shapiro says, is what made him switch to engineering after studying neuroscience as an undergraduate at Brown University in Providence, R.I. He realized that existing tools for studying processes inside the brain fell short. “And I didn’t see enough people making better tools.”
But he didn’t stop at developing new neuroscience technologies. “Oddly enough, once I got into the engineering part of things, I got so fascinated with weird proteins, and magnetic fields, and sound waves, and all the more physics-y side of things. That’s become as much, if not more, of my passion as the original neuroscience.” In his Twitter bio, Shapiro describes his expertise as succinctly as possible: “Bio-Acousto-Magneto-Neuro-Chemical Engineer at Caltech.” — Laura Sanders
Regeneration through an engineer’s eyes
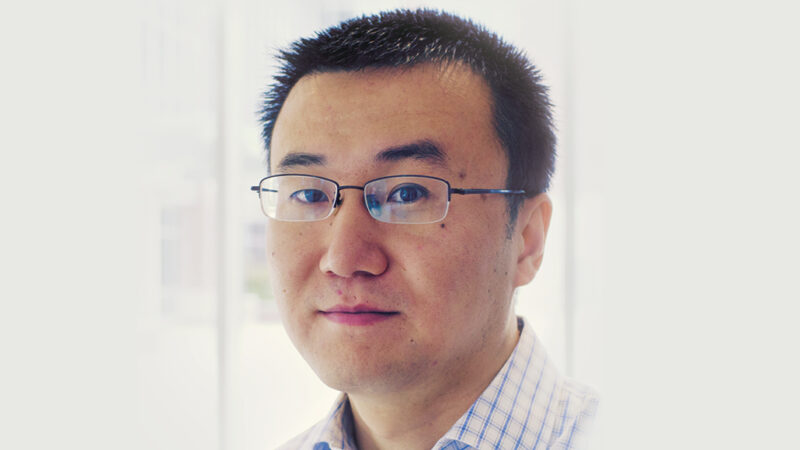
Bo Wang, 39Bioengineer
Affiliation: Stanford UniversityHometown: Nanjing, ChinaFavorite organism: Planarian
Inspiration
Planarians are the most charismatic of all flatworms, Bo Wang says. “They have this childish cuteness that people just love.” But the adorable facade isn’t what drew Wang to study the deceptively simple worms, which resemble little arrows with eyes. It was planarians’ superpower: regeneration. Slice a planarian into pieces and, within a week or two, each chunk will grow into a new flatworm — head and all. Studying the cells that drive this process could offer lessons for turning on regeneration in human tissues, to treat various diseases, regrow limbs and grow organs for next-generation transplants.
Bold idea
Wang uses statistical physics to figure out how planarians regenerate entire organs cell by cell. Newly formed brain cells, for instance, must physically position themselves to avoid turning into “amorphous aggregates,” Wang says. His interest in how things fit together began in graduate school at the University of Illinois at Urbana-Champaign. There, Wang trained as a physicist and worked on self-assembling materials. Wang now works to uncover the physical rules that living cells follow. “I’m fascinated by how molecules arrange themselves seemingly randomly, but there are still statistical rules that those molecules will follow,” he says.
Bo Wang works to uncover the physical rules that living cells follow.
His physics-based approach is raising new questions and unveiling biological processes that would be hard for biologists to come by using traditional methods alone, says regeneration biologist Alejandro Sánchez Alvarado of the Stowers Institute for Medical Research in Kansas City, Mo. Wang is “a new breed” of flatworm biologist, Sánchez Alvarado says. “He is occupying a very unique niche in the community of developmental biology.”
Standout research
Wang and colleagues recently found that nerve cells, or neurons, in regenerating planarian brains form a predictable pattern dictated by the types of cells in their midst. Planarians brains are akin to cities made up of neighborhoods of neurons. Within each neighborhood, no two neurons that do the same job will live next to each other; those cells repulse each other but stay close enough to communicate, the researchers reported in the May Nature Physics. Because of this behavior, increasing the types of neurons in a neighborhood limits the ways cells can pack together. The team dubbed this packing process “chromatic jamming,” after a famous mathematical puzzle called the four-color problem (SN: 3/6/09).
The finding is surprising and challenges “what we think we understand about organogenesis and about organization of cells within an organ,” says Sánchez Alvarado. Chromatic jamming appears to be key to how the planarian brain comes together, guiding single cells into neighborhoods that are a driving force in organ development, he says. If similar physical rules apply to human cells, that could help scientists sketch blueprints for engineering and growing artificial organs. — Cassie Martin
Cheaters can’t evade this genetic sleuth
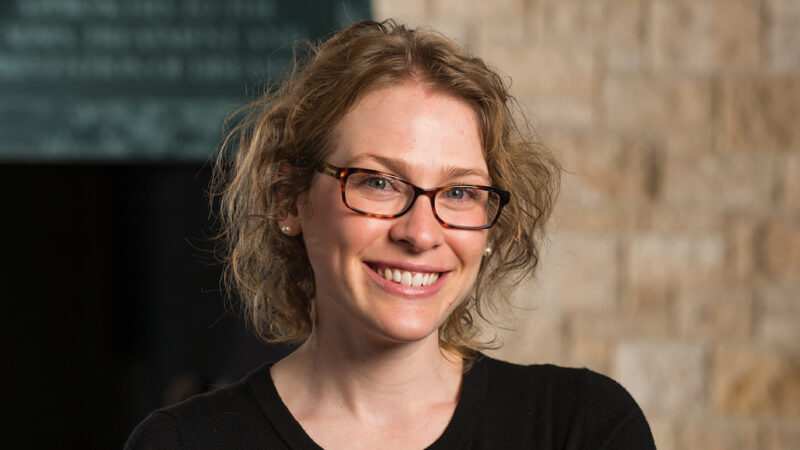
SaraH Zanders, 37Geneticist
Affiliation: Stowers Institute for Medical ResearchHometown: Glenwood, IowaFavorite organism: Fission yeast
Backstory
An invitation to work in the lab of her genetics professor Robert Malone at the University of Iowa in Iowa City set SaraH Zanders on the path to becoming a scientist. “It was a turning point in my life,” Zanders says. Before that, she didn’t really know how she would put her biology degree to use, or what it meant to be a scientist. In Malone’s lab, she fell in love with meiosis, the process by which organisms divvy up genetic information to pass on to future generations. The first step is julienning the genome and swapping pieces of chromosomes. “That just seems like such a bad idea to basically shred your [DNA] in the process of getting it from one generation to the next,” she says. She started studying the proteins involved in making the cuts. “It was like I was born to do that. I never would have known without that push.”
A different kind of push led Zanders to spell her first name with a capital H: An elementary school teacher kept leaving the letter off. Zanders has capitalized it for emphasis ever since. “If I write it without the big H, it doesn’t look like my name anymore,” she says. “It feels like somebody else.”
Standout research
Meiosis is full of conflict. For her postdoctoral work, Zanders focused on a particular type of dustup caused by some selfish genes — genes that propagate themselves even if it hurts the host. As the monk Gregor Mendel laid out in his study of pea plants, a particular version of a gene typically has a 50-50 chance of being passed on to the next generation. But the selfish genes Zanders was studying, a type called meiotic drivers because they propel themselves during meiosis, manage to get themselves inherited far more often. “These kinds of systems do a complete end run around Mendel’s laws,” says Daniel Barbash, an evolutionary geneticist at Cornell University.
In Schizosaccharomyces pombe, also called fission yeast, Zanders discovered, a family of selfish genes makes moves that would be right at home in a Game of Thrones story line. Zanders and colleagues were the first to work out the molecular tricks that thesegenes use to skirt Mendel’s laws, reporting the findings in eLife in 2017. The genes, known as wtf genes, produce both a poison and an antidote. All of the spores — the yeast’s gametes — get the poison, but only those that inherit certain gene versions also get an antidote. Spores that don’t get the antidote die, ensuring that only offspring with specific wtf gene versions survive to pass their genes on to the next generation. For the fission yeast, such predatory tactics can have big consequences, even driving two nearly identical strains toward becoming different species. Some selfish genes have made themselves essential for proper development (SN: 7/3/18). In humans and other animals, genetic conflicts may lead to infertility.
For the fission yeast, such predatory tactics can have big consequences, even driving two nearly identical strains toward becoming different species.
“This extremely important family of meiotic cheaters has been just sitting in plain sight waiting for somebody who had the right kind of lens and the care … to discover them,” says Harmit Malik, an evolutionary geneticist at the Fred Hutchinson Cancer Research Center in Seattle and Zanders’ postdoctoral mentor. Zanders helped build a case that the skewed inheritance in these yeast was a real effect, not just fluctuations in the data. Before she began her work, virtually nothing was known about meiotic drivers in yeast. Now the wtf genes are among the best known meiotic drivers studied in any lab organism. Some selfish genes in worms also use the poison-antidote trick to beat the competition (SN: 5/11/17). Meiotic drivers in fruit flies, mice — and maybe humans — win genetic conflicts by other means (SN: 10/31/17; SN: 2/24/16).
Motivation
Zanders is now on the lookout for other genetic fights in yeast. Understanding such conflicts more generally may help answer big questions in evolution, as well as shedding light on human infertility. As for what motivates her, “It’s just I like to solve puzzles,” Zanders laughs. “I wish it was a deep desire to help people, but it’s definitely not that.” — Tina Hesman Saey
Quake expert co-opts underground cables
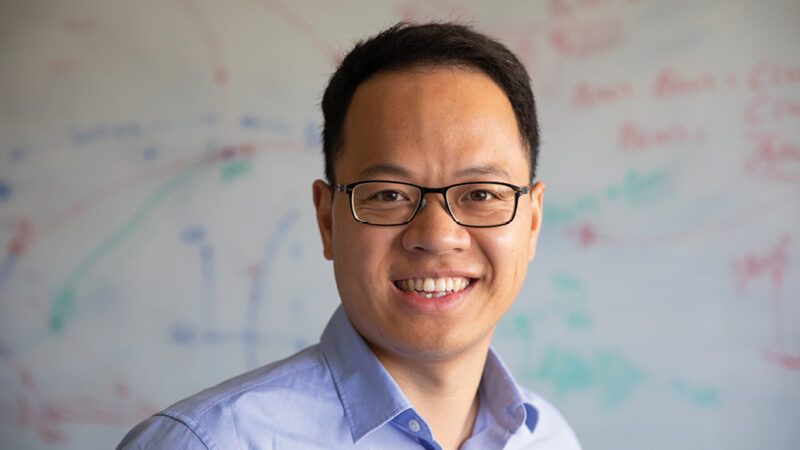
Zhongwen Zhan, 33Seismologist
Affiliation: CaltechHometown: Jinzhai County, ChinaFavorite hobby: Carpentry
Big goal
As the Rose Parade wound through Pasadena, Calif., on January 1, 2020, Zhongwen Zhan listened to the underground echoes of the marching bands and dancers. With a sensitive technology known as distributed acoustic sensing, or DAS, Zhan tracked the parade’s progress. He even identified the most ground-shaking band. (It was the Southern University and A&M College’s Human Jukebox.)
The study was a small but elegant proof of concept, revealing how DAS is capable of mapping out and distinguishing among small seismic sources that span just a few meters: zigzagging motorcycles, the heavy press of floats on the road, the steady pace of a marching band. But Zhan seeks to use the technology for bigger-picture scientific questions, including developing early warning systems for earthquakes, studying the forces that control the slow slide of glaciers and exploring seismic signals on other worlds.
Zhan has a “crystal-clear vision” of DAS’ scientific possibilities, says Nate Lindsey, a geophysicist at Stanford University who is also part of the small community of researchers exploring the uses of DAS. “When you get such a cool new tool, you like to just apply it to everything,” he adds. But Zhan’s expertise is “very deep, and it goes into many different areas. He knows what’s important.”
So far, Zhan and other researchers have used the technology to study aftershocks following the 2019 Ridgecrest earthquakes in Southern California (SN: 7/12/19), to demonstrate that interactions between ocean waves produce tiny quakes beneath the North Sea, and to examine the structure of glaciers.
Top tool
DAS piggybacks off the millions of fiber-optic cables that run beneath the ground, ferrying data for internet service, phones and televisions (SN: 6/14/18). Not all of the glass cables are in use all of the time, and these strands of “dark fiber” can be temporarily repurposed as seismic sensors. When pulses of light are fired into the fibers’ ends, defects in the glass reflect the light back to its source. As vibrations within the Earth shift and stretch the fibers, a pulse’s travel time also shifts.
Whole networks of seismic sensors could be deployed in places currently difficult or impossible to monitor — at the ocean bottom, atop Antarctic glaciers, on other planets.
Over the last few years, scientists have begun testing the effectiveness of these dark fibers as inexpensive, dense seismic arrays — which researchers call DAS — to help monitor earthquakes and create fine-scale images of the subsurface. In these settings, Zhan notes, DAS is proving to be a very useful supplement to existing seismograph networks. But the potential is far greater. Whole networks of sensors could be deployed in places currently difficult or impossible to monitor — at the bottom of the ocean, atop Antarctic glaciers, on other planets. “Seismology is a very observation-based field, so a seismic network is a fundamental tool,” he says.
Inspiration
“I’ve been interested in science since I was young, but wasn’t sure what kind of science I wanted to do,” Zhan says. In China, students usually have to decide on a field before they go to college, he adds, but “I was fortunate.” At age 15, Zhan was admitted to a special class for younger kids within the University of Science and Technology of China in Hefei. The program allowed him to try out different research fields. A nature lover, Zhan gravitated toward the earth sciences. “Environmental science, chemistry, atmospheric science — I tried all of them.”
Then, in late 2004, a magnitude 9.1 earthquake ruptured the seafloor under the Indian Ocean, spawning deadly tsunamis (SN: 1/5/05). After hearing from a researcher studying the quake, Zhan knew he wanted to study seismology. “I was amazed by how seismologists can study very remote things by monitoring vibrations in the Earth,” Zhan says. The data “are just wiggles, complicated wiggles,” but so much info can be extracted. “And when we do it fast, it can provide a lot of benefit to society.” — Carolyn Gramling